Space nuclear propulsion: Humanity’s route to the solar system
Part II: Electric propulsion and fission power generation in space
(Part I, "Space nuclear reactor safety," is here)
Ever since man set foot on Earth's moon, explorers have envisioned traveling out of Earth's orbit and into space beyond. To do so, however, will require a propulsion device capable of traveling farther than any used before. These devices will be powered by nuclear energy. In this post, I will discuss nuclear electric propulsion, one of the two primary nuclear propulsion concepts considered by engineers for near-term space travel. Nuclear thermal propulsion, the other primary concept, will be explored in a later post.
Electric propulsion, also commonly referred to as "ion thrust propulsion," uses electrical power to accelerate ions to very high speeds to provide thrust for a spacecraft. Nuclear electric propulsion is electric propulsion whose power source is fission reactor based, or radioisotope decay based. Electric propulsion is not a new technology, and is well understood. Currently, solar powered electric propulsion devices are used at a small scale to keep satellites in their correct orbit. For operation at a larger scale, however, where much higher thrust values will be needed, or operation at a distance from the sun where solar power is incapable of providing the necessary energy intensity, nuclear electric propulsion will be required.
Aside from the ion thruster used to provide thrust for a nuclear electric propelled spacecraft, its other defining characteristic is the type of nuclear electric generator needed to provide power. Nuclear electric generators used for power production in space can come in many shapes and sizes, depending on the power requirements and spacecraft dimensions. For small power production needs in missions requiring a low thrust, radioisotope power systems can be used. In these systems, the radioactive decay heat from a radioisotope is converted to electricity through the use of a heat-to-work conversion device, or a heat engine. The most common of these are Radioisotope Thermoelectric Generators (RTGs) and Advanced Stirling Radioisotope Generators (ASRGs). You can find out more about these systems by reading my previous post on plutonium-based radioisotope power systems.
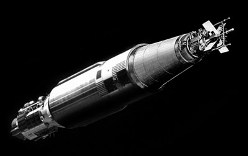
SNAP 10-A, fission-based space power system launched in 1965
For larger thrust requirements, however, fission-based power systems become a necessity. Missions requiring such high thrust will be manned and/or carry a large onboard capacity for conducting science. These mission requirements are also often outside the capability of chemical (or even nuclear thermal) propulsion. Individually, the previously stated requirements are not difficult to meet. For example, earth-based power reactors generate enough power to light a large city, but the thought of launching them into space to produce power is absurd. Alternatively, fission power systems have been proposed that are about the size of a small car, which is a relatively small payload to put into low earth orbit. Unfortunately, these systems can produce only a fraction of the electric power that could be produced by that same small car. The ideal space nuclear electric generator would meet both requirements of size and power. To evaluate competitive designs for nuclear electric propulsion systems, engineers seek the smallest system mass possible for a given power production level.
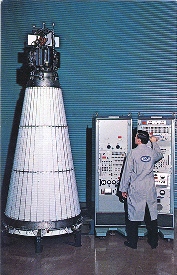
SNAP 10-A
To meet these system requirements, engineers must consider different technologies from those used in earth-based nuclear reactors. For example, the first and only fission-based space power system to be flown by the United States, the SNAP-10A spacecraft, used thermoelectrics, which is the same power conversion technology used by RTGs to produce electricity. Thermoelectrics, however, while dependable, are very inefficient, and excess heat produced by the reactor must be rejected away from the spacecraft. In space, this heat rejection can only be in the form of radiative energy. For those unfamiliar with methods of heat transfer, radiative heat transfer is how heat lamps heat food at a local fast food restaurant, how heat is lost from a vacuum sealed Thermos, and even how the earth is heated by the sun. This may seem unintuitive at first but if you think about it, there are no lakes or rivers of water in space to sweep away excess heat like earth-based power systems. In space nuclear power systems, large panels are heated to high temperatures in order to reject this excess heat. Thus the size, and accordingly the temperature, of these radiator panels drive the power system to be as efficient and high temperature as possible.
Many technologies have been discussed as being capable of achieving such power production goals, with some being invented primarily for this purpose. One of the simpler systems may use a combination of helium and xenon gas as coolant, which can spin a turbine to produce electricity. This was the system designed and proposed for use in the Jupiter Icy Moons Orbiter (JIMO), a space exploration program under serious consideration only a few years ago. More complicated systems propose boiling potassium to spin a turbine, although the zero gravity environment of space makes the task more difficult to accomplish. Lastly, some propose suspending the fuel in a gaseous form, allowing it to flow through a magnetohydrodynamic generator (MHD), which uses the ionized fuel particles to produce electricity. The best way to explain an MHD generator is to think of it as a reverse ion thruster, where charged particles induce a current to produce electricity.
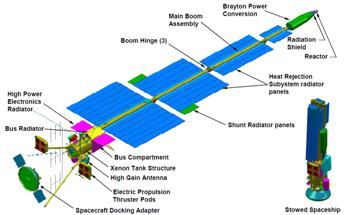
Prometheus nuclear electric Deep Space Vehicle, incorporating JIMO Mission Module
Nuclear electric propulsion has great potential. Its ability to provide propulsion to anywhere in the solar system makes it a viable competitor when the human race decides to explore beyond the gravity well of earth. Like most nuclear technologies, research will continue and technological advancements will continue to be made in the meantime.
_________________________
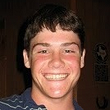
Deason
Wes Deason is a graduate student in nuclear engineering at Oregon State University working on the safety analysis of vented fuel systems for gas-cooled fast breeder reactors. He is a former summer fellow for the Center for Space Nuclear Research and the current student liaison for the Aerospace Nuclear Science and Technology Division of the American Nuclear Society.