Update and Perspective on Small Modular Reactor Development
The US Department of Energy has a $452 million program to share development and licensing costs for selected small modular reactor (SMR) designs. The DOE's goal is to have an operating SMR by ~2022. Last November, the DOE awarded the first grant to the B&W mPowerTM reactor. In more recent news, the DOE has decided to issue a follow-on solicitation to enter a similar cost-sharing agreement with one or more other SMR vendors (and their SMR designs). The status of development and licensing for several SMR designs are summarized below.
mPower (B&W)
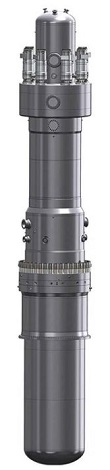
B&W mPower SMR
The mPower reactor is a 180-MW pressurized water reactor. B&W was awarded the first cost-sharing agreement under the DOE's SMR development program in November 2012. B&W has teamed up with Bechtel and the Tennessee Valley Authority to design, license, and build a set of 2-6 mPower modules at TVA's Clinch River site. B&W plans to submit its design certification application (DCA) to the Nuclear Regulatory Commission by the end of this year.
NuScale
The NuScale reactor is an even smaller, 45-MW PWR reactor module. NuScale Power will apply for the follow-on (second round) DOE program cost-sharing award that was just announced. It has partnered with Fluor to develop and build the SMR, and is considering building its first SMR modules at the DOE Savannah River Site (SRS). It expects to submit its DCA to the NRC some time in 2015.
Holtec
Holtec International, which is developing a 160-MW (light water) SMR, may also apply for the second DOE grant, and is also interested in constructing its SMR at the SRS site.
Westinghouse
Westinghouse is developing a 225-MW PWR that shares many design features of its larger AP1000 plant. It is partnering with Burns & McDonnell, Electric Boat, and the Ameren utility to design, license, and build its first SMR plant at Ameren's existing Callaway plant site in Missouri. It is expected to also apply for the second round of cost-sharing grants under the DOE's SMR program. Westinghouse is expected to submit its DCA to the NRC in 2014.
Non-LWR SMRs
The most advanced non-light water SMR project is the Gen4 Energy's lead-bismuth-cooled 25-MW reactor module (formerly Hyperion). Given the DOE's focus on near-term SMR deployment, however, and the NRC's indication that licensing a non-LWR will take a much longer amount of time, it is unclear whether non-light water SMRs have much prospect for winning a cost-sharing award under the DOE's current SMR development program. Gen4 Energy withdrew its application for the initial round of DOE grants and it is not clear if it will apply for the second round.
Key desirable SMR features
My personal view is that SMRs should (ideally) have the following three features, entirely or to the extent possible:
- The entire nuclear steam supply system (NSSS) can be factory built and rail-shipped to site.
- The reactor can go indefinitely without offsite power or forced (pumped) cooling.
- No on-site construction subject to NQA-1 requirements.
In a recent ANS post, I discussed issues such as the basemat rebar (and other) problems at Vogtle, as an example of the problems that are likely to occur when there are a large number of construction activities that are subject to NQA-1 and NRC oversight being performed on site, often by local suppliers or craft labor that do not have extensive experience with nuclear-related construction. Processes are much more controlled in a factory setting, where one is simply making a large number of copies of the exact same product (reactor design). Also, the factory would have dedicated staff that is highly experienced in making copies of that one product, and is very experienced with the applicable nuclear-grade fabrication and quality assurance requirements (e.g., NQA-1). The result is much higher levels of quality and consistency, with much less in the way of cost overruns or schedule delays.
For these reasons, it is imperative to have as much of the safety/nuclear-related construction as possible be done at the factory, and to minimize assembly and construction activities at the plant site. Thus, it is very preferable to have the entire NSSS (reactor and steam supply system, e.g., steam generators) sealed inside a container that can be shipped by rail to the plant site, without any at-site assembly required. Ideally, all components necessary for safety would be inside the "box" that arrives on the rail car, resulting in only non-nuclear grade construction activities at the site.
In that recent ANS post, I suggested that due to spiraling nuclear plant construction costs, a bottoms-up review is in order, in which all regulations and requirements are put on the table and objectively evaluated (using detailed probabilistic risk analyses, etc.) in terms of how much "bang for the buck" we're getting in terms of overall safety. I made the suggestion (provocative to many, I'm sure) that NQA-1, i.e., a unique and extremely strict set of fabrication/QA requirements that only applies to the nuclear industry, most likely does not produce much safety benefit relative to its associated cost. I suggested that more typical QA standards and procedures that are used in most other large construction projects (bridges, dams, etc.) be used instead.
Well, with SMRs a "compromise" may be possible. Based on recent experience with Areva's EPR (at Olkiluoko) and now at Vogtle, I had come to doubt that it was possible or practical to comply with those NRC and NQA-1 requirements, with on-site plant construction anyway. It seemed to be too difficult to comply with such strict requirements under field conditions, especially given the use of local labor and suppliers that do not have extensive experience with those requirements. The factory assembly line setting, however, is one setting where I can imagine it being practical to comply with strict NRC/NQA-1 requirements (with highly experienced staff, a controlled process, and NRC inspectors permanently present at the factory site).
Thus, with SMRs, almost all important-to-safety fabrication is performed at the factory site, and it could still be held to NQA-1 standards. Onsite activities at the nuclear plant that are subject to NQA-1 requirements can be greatly reduced or perhaps (as part of a "compromise") eliminated. In my view, not having onsite construction activities be subject to (nuclear-unique) NQA-1 requirements, and instead letting the local construction entities use more typical QA requirements that they are familiar with, would greatly reduce costs and the risks of schedule delays, rework, and cost overruns. On the other hand, having NQA-1 standards apply at the reactor module factory would deliver virtually all of NQA-1's safety benefit, without significantly increasing costs.
Finally, it would be highly desirable for the plant to have the attribute of always remaining sufficiently cool to avoid meltdown for an indefinite period without any outside power or active cooling (pumps, etc.). Post-Fukushima, such a feature may greatly increase political and public support for the reactor design. Also, such a feature would greatly reduce the plausible conditions under which meltdown and release could possibly occur. This, in turn, could greatly reduce the number of components or systems that must be classified as "safety related", which would result in significant cost reductions (as well as reductions in actual accident/release probability).
Features of SMR candidates
The main SMR candidates that meet the goals listed above are as follows, based on their publicly presented information:
The mPower and NuScale vendors state that their entire NSSS will be fabricated at the factory and shipped (whole) to the plant site. Westinghouse is less clear, referring to "rail shippable scale" (which could refer to the entire NSSS, or a small number of NSSS component modules, which would require a limited amount of on-site assembly).
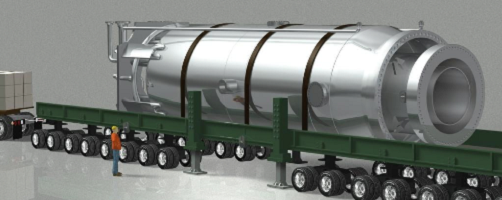
Hauling the NuScale reactor
NuScale very clearly states that its SMR is entirely passively cooled, and can go indefinitely without outside power and active (pumped) cooling. B&W (mPower) is less clear on this point, stating that no AC power is required for design basis safety functions, that they have three-day batteries to support DC-powered accident mitigation, and that the station can go up to 14 days (under loss of power conditions) without outside intervention. Gen4 Energy also states that its (lead-bismuth) reactor can go 14 days without power. I could not find a statement from Westinghouse concerning how long its SMR can go without any external power. Westinghouse does make reference to the operator having to add water (to a large tank) after seven days.
As expected, none of the SMR vendors discuss fabrication QA requirements for at-plant-site construction and components, or how many such components would be classified as safety related. Some have, however, performed some PRA analyses and do discuss the very low probability of core damage and significant release for their reactors. B&W (mPower) and NuScale state that their core damage frequencies (CDFs) are 10-8 and 10-7 per reactor year, respectively. By comparison, currently operating plants generally have CDFs of ~10-4 per reactor year and more recent large plants (e.g., AP1000) have CDFs under 10-6.
Cost and safety tradeoffs
Due to their smaller size and lower power densities, SMRs offer inherent safety advantages, largely because smaller reactors are easier to keep cool. As shown above, their chances of core damage are far lower than those of large reactors. In addition to a lower probability of core damage, their much smaller fuel inventory greatly reduces the maximum possible release. In fact, since these reactors probably can't get nearly as hot, even in a core damage scenario, I'm guessing that their maximum core inventory release fractions (e.g., for cesium) under even worst-case meltdown conditions are also significantly smaller than those that apply for larger reactors. Thus, the maximum possible release is probably even less than the ratio of reactor powers (MW) would imply.
In order to get these advantages (along with the advantages of assembly line construction), they have to give up on economy of scale and power density, which will tend to increase costs. Some SMR vendors claim that groups of their modules will produce less expensive power than large reactors (e.g., the AP1000), but this remains to be seen. It is also unclear whether these modular reactors will be less expensive than fossil fuels (particularly gas). As I've often stated, these reactors cannot provide any health, environmental, or global warming benefits if they are not deployed. Thus, some actions may need to be taken to reduce costs.
This leads me to ask what SMRs will "get in return" for what they are giving up in terms of scale, power density, and increased fundamental safety. We may have to ask if there are any measures that could be taken that would reduce costs but result in a release probability that is closer to that of, say, the AP1000, as opposed to being orders of magnitude lower. In these evaluations, the much lower potential release from these reactors should also be fully considered. I believe that thorough evaluations of all potential cost-saving measures, supported by detailed PRA evaluations, should be performed.
One idea I discussed earlier is to use ordinary construction QA requirements for all on-site construction activities (i.e., for everything outside the NSSS that arrives by rail car). Given the much lower likelihood of core damage/release, the much smaller potential releases, and the fact that components outside the NSSS have a relatively small impact on overall safety (especially for these reactors), such an approach would be justified. In evaluating such an approach, we need to make reasonable determinations of both the probability and possible nature of failures of non-nuclear-qualified components. For example, the NuScale reactors lay in a large pool of water inside a concrete-walled underground pit. We have to ask ourselves: Is there any way the concrete could fail that would result in the water disappearing (especially given that the pool is underground)?
Other issues are operator and security staffing levels. The simplicity and inherently better safety of these designs should reduce the number of required operators and staff (and some SMR vendors are claiming just that). Security costs could be greatly reduced (in my view) if SMRs are placed on existing sites where large reactors already exist. Little extra security should be required, since the site is already protected.
Also, as discussed in my earlier post, licensing review should be fairly limited if one is placing a certified SMR design on a site that already has reactors. Almost like spent fuel dry storage casks, a simple review of the existing site evaluations, to verify that external parameters such as maximum ground accelerations and other environmental factors are bounded by the SMR's generic safety evaluations, should be sufficient. An evaluation of some bounding number of reactor modules would then be done to address any impacts of the reactors on the site (e.g., a site boundary dose evaluation). After that is done, modules could be added without further licensing activity.
The NRC's general philosophies, however, as well as some of its recent actions, leads me to believe that any kind of compromise may be too much to expect. In response to Fukushima, the NRC is increasing nuclear regulations even further. While we all agree that some specific improvements can and should be made as a result of lessons learned from Fukushima, there has been absolutely no discussion at all about whether any requirements should be pared back. This, despite the fact that Fukushima showed that the consequences of a severe (almost worst-case) meltdown are FAR smaller than what we had thought (and far smaller than the assumed accident consequences that many if not most of those requirements were based upon). For this reason, I'm inclined to believe that the NRC will take all the benefits of SMRs (i.e., the great reduction in release probability due to fundamental features) and give absolutely nothing back. That, despite the fact that some economic sacrifices (on economy of scale) had to be made in order to get those fundamental increases in safety.
If SMRs are to be viable, and provide safety, health, environmental, and global warming benefits, the NRC is going to have to make some compromises. If they do, SMRs may be able to provide an option that is not only economically competitive (allowing it to displace harmful fossil fuels), but is also far safer than current US nuclear plants, and as safe or safer than new large plants such as the AP1000.
_______________________
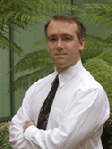
Hopf
Jim Hopf is a senior nuclear engineer with more than 20 years of experience in shielding and criticality analysis and design for spent fuel dry storage and transportation systems. He has been involved in nuclear advocacy for 10+ years, and is a member of the ANS Public Information Committee. He is a regular contributor to the ANS Nuclear Cafe.