Neutron Vision at Los Alamos: Exploring the Frontiers of Nuclear Materials Science
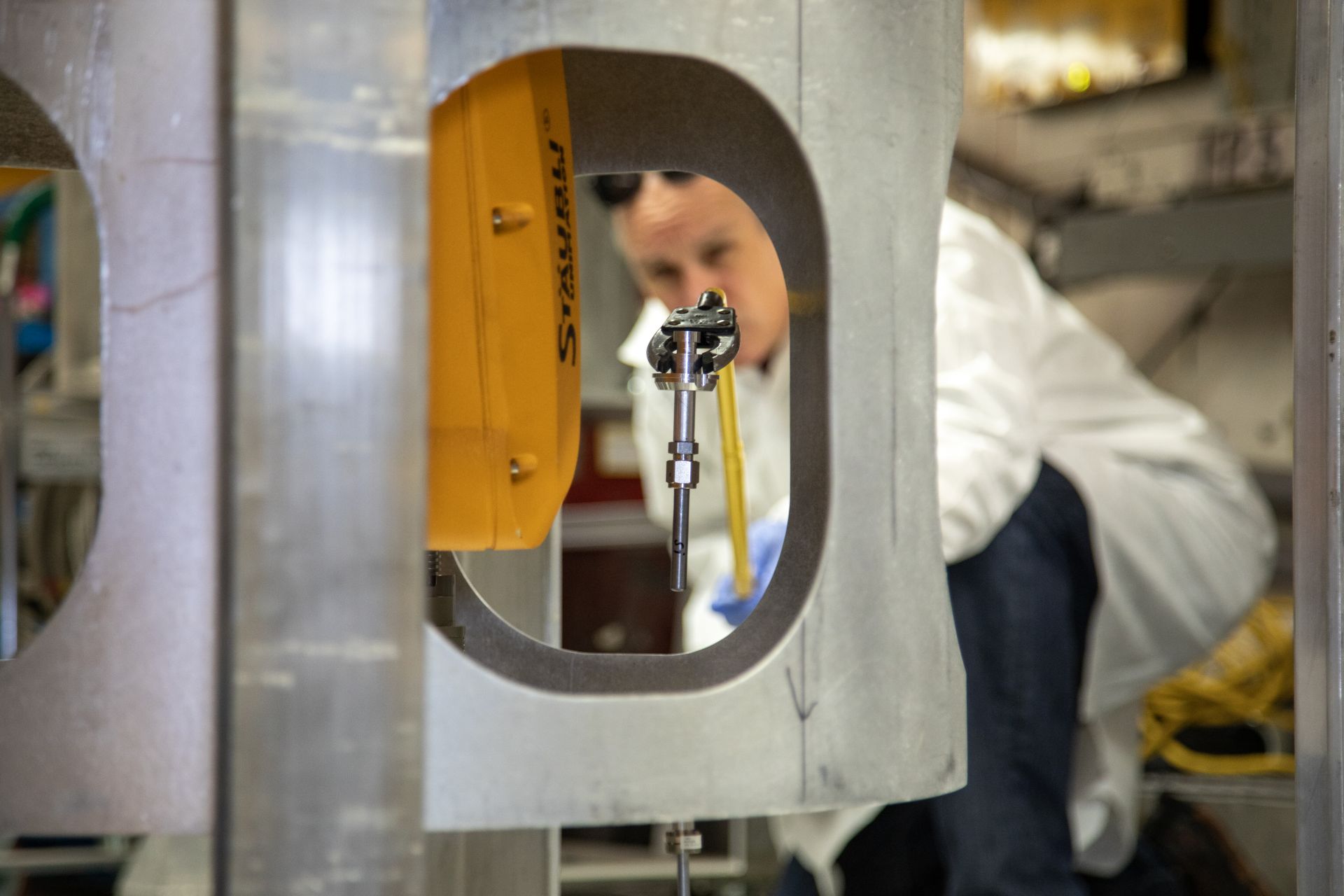
In materials science, understanding the unseen—how materials behave internally under real-world conditions—has always been key to developing new materials and accelerating innovative technologies to market. Moreover, the tools that allow us to see into this invisible world of materials have often been game-changers. Among these, neutron imaging stands out as a uniquely powerful method for investigating the internal structure and behavior of materials without having to alter or destroy the sample. By harnessing the unique properties of neutrons, researchers can uncover the hidden behavior of materials, providing insights essential for advancing nuclear materials and technologies.
Characterizing nuclear materials: Why neutrons, why imaging, and why now?
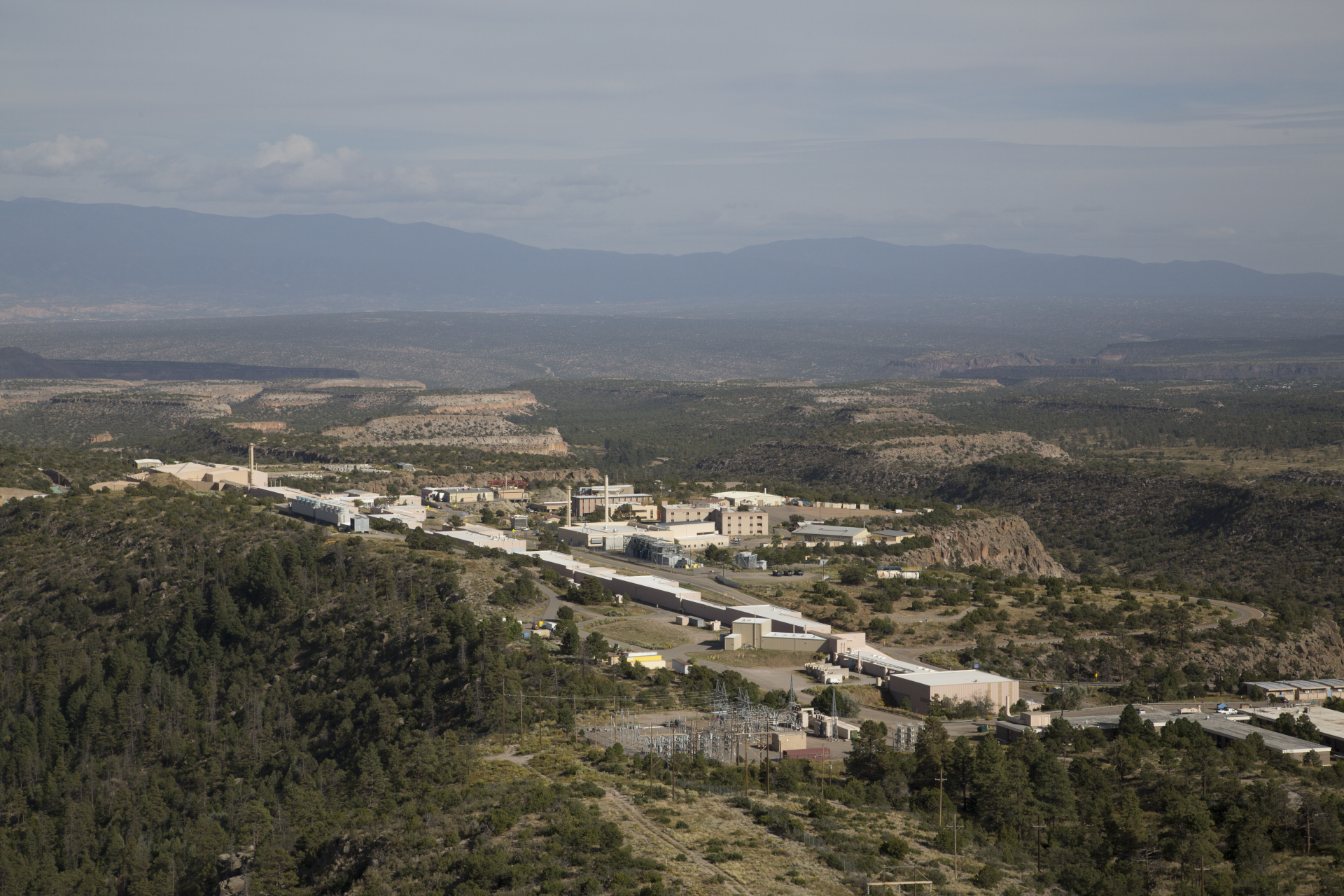
An aerial view of the Los Alamos Neutron Science Center (LANSCE), showcasing the front end of the accelerator complex nestled atop the scenic mesas of northern New Mexico. (Photo: LANL)
Neutrons are exceptional tools for probing material properties, offering unique advantages compared to other techniques. Unlike X-rays, which interact with electron shells, neutrons interact with atomic nuclei. This fundamental difference creates a complex and fascinating landscape for generating contrast in terms of imaging. More specifically, neutron interactions do not follow the predictable trends observed with X-rays or protons, as they are highly isotope-dependent and exhibit significant variations as a function of neutron energy for any given isotope. This allows neutrons, in general, to penetrate dense, heavy materials with ease while remaining remarkably sensitive to lighter elements, such as hydrogen. This combination of deep penetrability, sensitivity to light elements, and energy-dependent interactions makes neutrons unique for studying materials in nuclear systems. Neutrons are particularly effective for probing materials under extreme conditions. Whether conducting in situ measurements at high temperatures or analyzing irradiated samples requiring heavy shielding, neutrons offer unique insights that are essential for advancing our understanding of nuclear materials and provide reliable data needed for simulations and ultimately licensing of nuclear reactors.
Neutron scattering techniques like diffraction have been invaluable for studying materials critical to nuclear energy, particularly for understanding their crystal structures and how they behave under extreme conditions. However, these techniques focus on bulk properties and cannot capture spatial variations, such as the distribution of crystallographic phases, material defects, or chemical and isotopic compositions within a sample. While bulk properties provide a strong foundation, developing materials for advanced reactors demands a deeper understanding of how properties change under extreme conditions. This need is particularly urgent as modeling and simulation tools become increasingly sophisticated, driving a growing demand for experimental data that captures spatially resolved material responses to validate and enhance their predictive capabilities.
Serendipitously, advancements in imaging technology over the past decade or so have enabled neutron imaging to meet the growing demand for more detailed and comprehensive characterization of nuclear materials. Improvements in detector sensitivity, spatial resolution, and acquisition modes have expanded neutron imaging’s capabilities, allowing researchers to visualize the internal structure and dynamics of materials with unprecedented clarity. These advancements have transformed neutron imaging from a qualitative method into a precise quantitative tool, allowing high-resolution mapping of microstructure and isotopic distributions—even in situ under extreme conditions with elevated temperatures or within a post-irradiation environment.
This level of understanding is not just a scientific endeavor—it is essential for ensuring the safety, efficiency, and longevity of next-generation reactors, which are set to play a pivotal role in the global transition to cleaner and more reliable energy. As next-generation nuclear reactor designs move closer to licensing and deployment, the need for a deeper understanding of the materials critical to their success is growing rapidly. These advanced reactors are designed to operate under more extreme conditions than traditional systems, including higher temperatures, corrosive environments, and greater radiation exposure. Addressing these challenges requires advanced characterization tools that can provide comprehensive insights into how materials respond and evolve under such demanding conditions.
Advanced neutron imaging for nuclear material characterization at Los Alamos
Los Alamos National Laboratory is renowned for its expertise in actinide research and materials science, which often involves handling highly hazardous materials in extreme environments, but its unique ability to combine cutting-edge neutron science with this type of capability is less well known beyond specialized research circles within the nuclear energy community. Home to the Los Alamos Neutron Science Center (LANSCE), the lab boasts a world class proton accelerator featuring two neutron spallation source user facilities: the Lujan Center (moderated neutrons) and the Weapons Neutron Research (WNR) Facility (unmoderated neutrons).
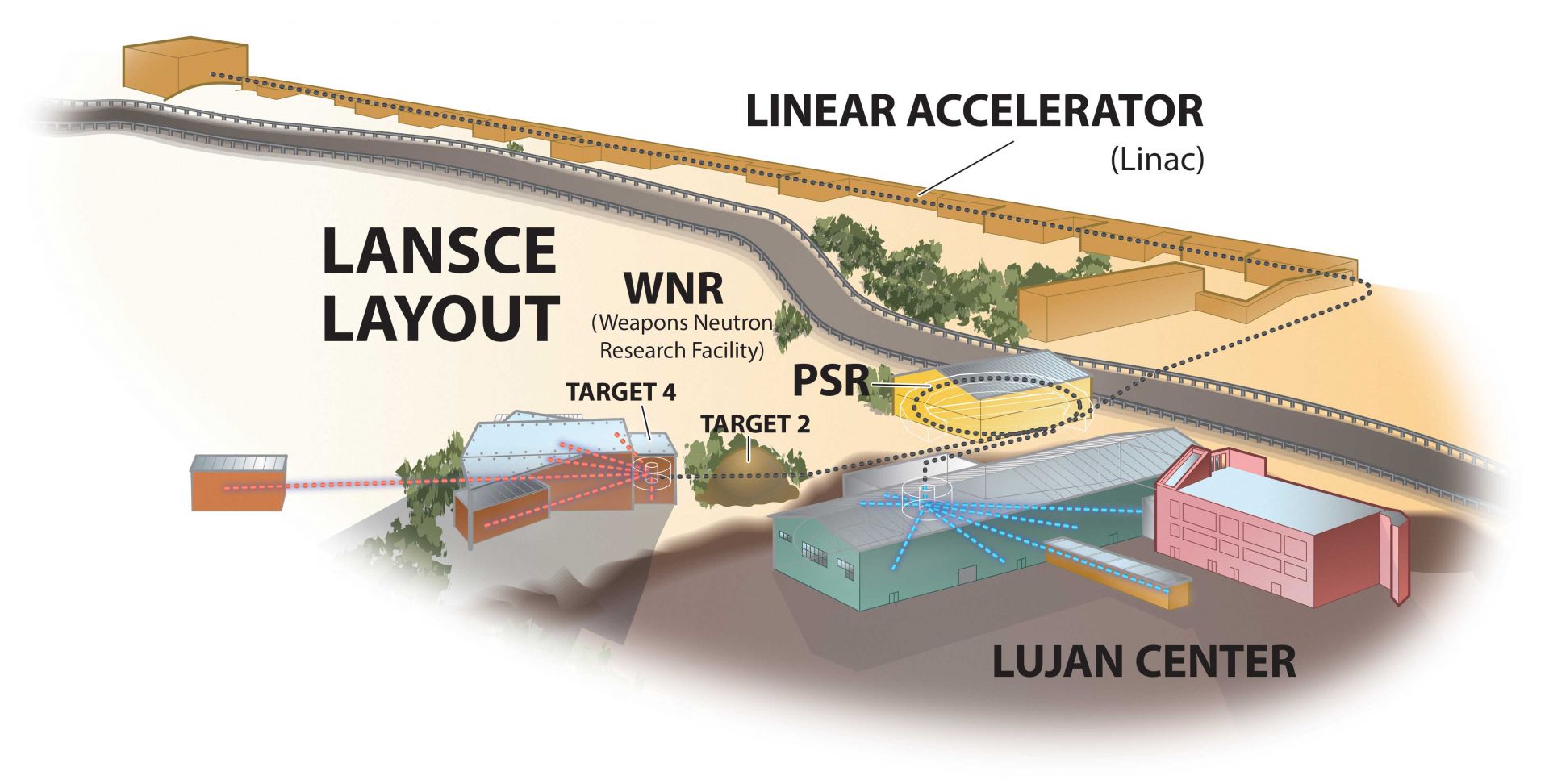
Layout of the two spallation neutron sources, the Lujan Center and the Weapons Neutron Research Facility, at LANSCE. (Image: LANL)
These spallation sources are driven by short proton pulses, which is a key feature enabling advanced neutron imaging techniques. By knowing the exact time neutrons are created and using advanced imaging detectors that time-tag when neutrons are detected, researchers can perform energy-resolved neutron imaging based on time-of-flight techniques. It is this method that allows researchers to leverage the unique, energy-dependent neutron cross sections to extract detailed isotopic or microstructure information on a pixel-by-pixel basis.
This capability is further enhanced by Los Alamos’s unique expertise and infrastructure for handling highly hazardous materials. At these facilities, researchers can apply these advanced neutron imaging techniques to study the material properties of actinides, such as uranium and plutonium, with unprecedented detail. These materials can be studied in a variety of forms—liquids, solids, or even post-irradiation—and under a wide range of extreme conditions. Together, these capabilities make LANSCE a unique hub for transformative materials science research in nuclear energy, accelerating innovation and expanding our understanding of materials crucial for advancing next-generation reactor designs.
At LANSCE, neutron imaging is carried out across multiple flight paths, each tailored to specific research needs and offering unique imaging modalities. At the Lujan Center, Flight Path 11, known as ASTERIX, specializes in cold neutron imaging, which excels in probing light elements and fine structural details within a given material. ASTERIX supports advanced techniques such as phase-contrast imaging and dark-field imaging using neutron grating interferometry, and hydrogen mapping based on traditional attenuation methods. Phase-contrast imaging enhances the visibility of interfaces and subtle density variations, while dark-field imaging provides insight into nanoscale structural variations, such as cracks, voids, and phase distributions. Hydrogen mapping leverages cold neutrons’ high sensitivity to hydrogen to quantify its distribution within a material, a capability critical for studying hydrogen migration, embrittlement, and other hydrogen-related phenomena in nuclear materials. Together, these modalities make ASTERIX a versatile and powerful neutron imaging flight path for advancing the understanding of material behavior under a wide range of conditions.
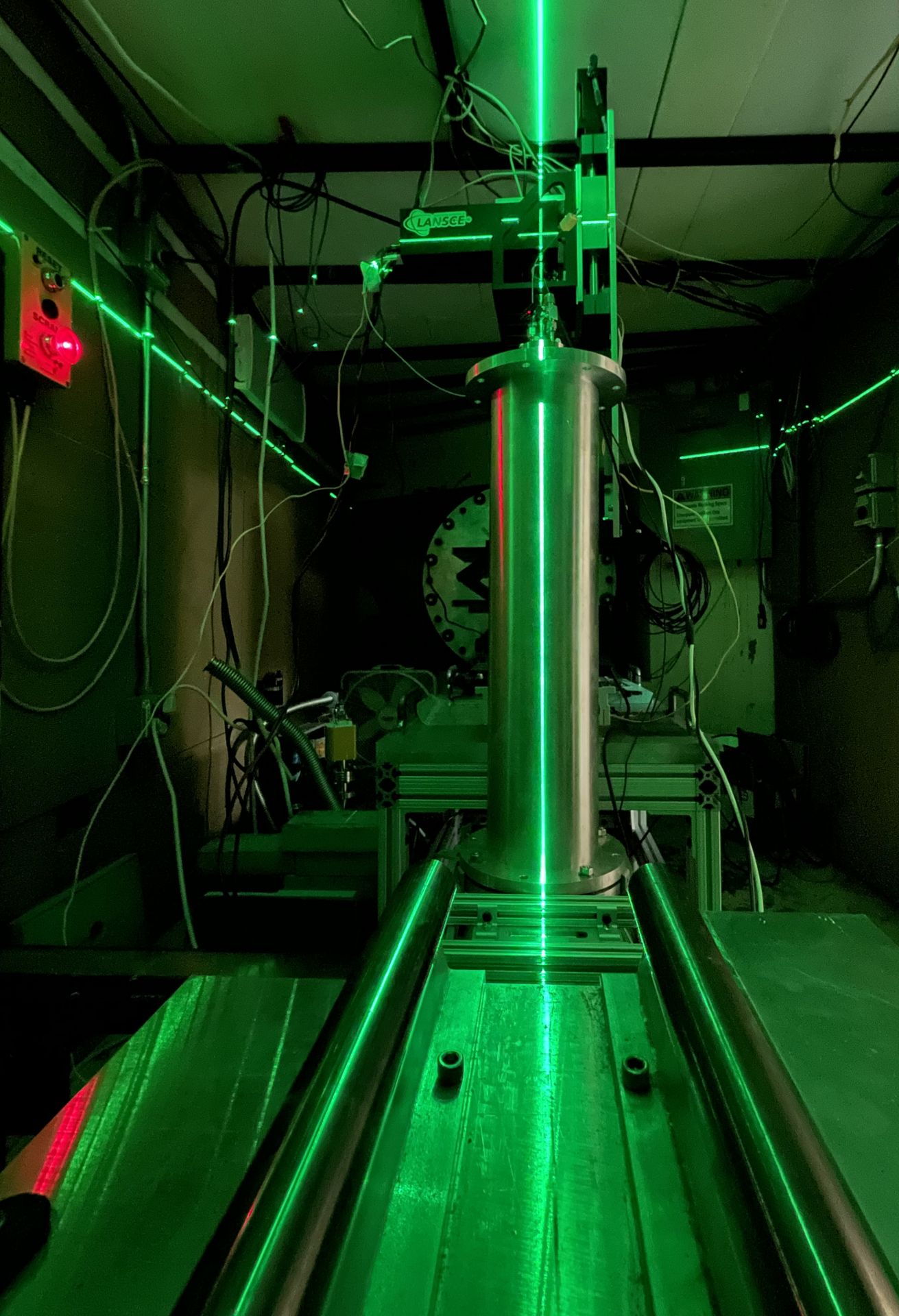
A custom-built compact imaging furnace on Flight Path 5 (ERNI) at the Lujan Center, designed for molten salt neutron imaging–based thermophysical properties measurements. This custom furnace can reach temperatures up to 1,200°C across a 12-inch-tall heating zone, enabling precise thermophysical measurements, such as density and viscosity, of molten salts. (Photo: LANL)
In addition to cold neutron imaging, LANSCE offers advanced thermal and epithermal neutron imaging capabilities on Flight Path 5 (ERNI) and Flight Path 4 (HIPPO) at the Lujan Center. These beamlines support techniques such as neutron resonance imaging (NRI) and Bragg-edge imaging (BEI), which excel at measuring isotopic and microstructure distributions in materials. NRI utilizes the unique energy-dependent absorption properties of neutrons to produce 2D maps of isotopic compositions. By employing computed tomography (CT) methods, NRI enables detailed 3D mapping of isotopic densities within a sample, providing insights into the internal composition with sub-millimeter resolution. This capability is particularly valuable for studying complex materials such as irradiated nuclear fuels, where understanding the distribution of isotopes like uranium and plutonium—along with other constituents—is essential for assessing performance and safety and for validating models. The nondestructive nature of imaging makes this technique especially effective for analyzing irradiated nuclear fuels, as it provides detailed isotopic maps even for samples enclosed in shielding containers. These 3D maps offer critical insights into the internal composition of the fuel, along with potential fission products, and can guide post-irradiation examination by identifying regions of interest for destructive analysis. By combining its ability to work with highly radioactive materials with its precision and nondestructive approach, NRI is a powerful material characterization tool and enhances the overall understanding of nuclear fuel behavior, especially when used as a supplemental post-irradiation examination technique.
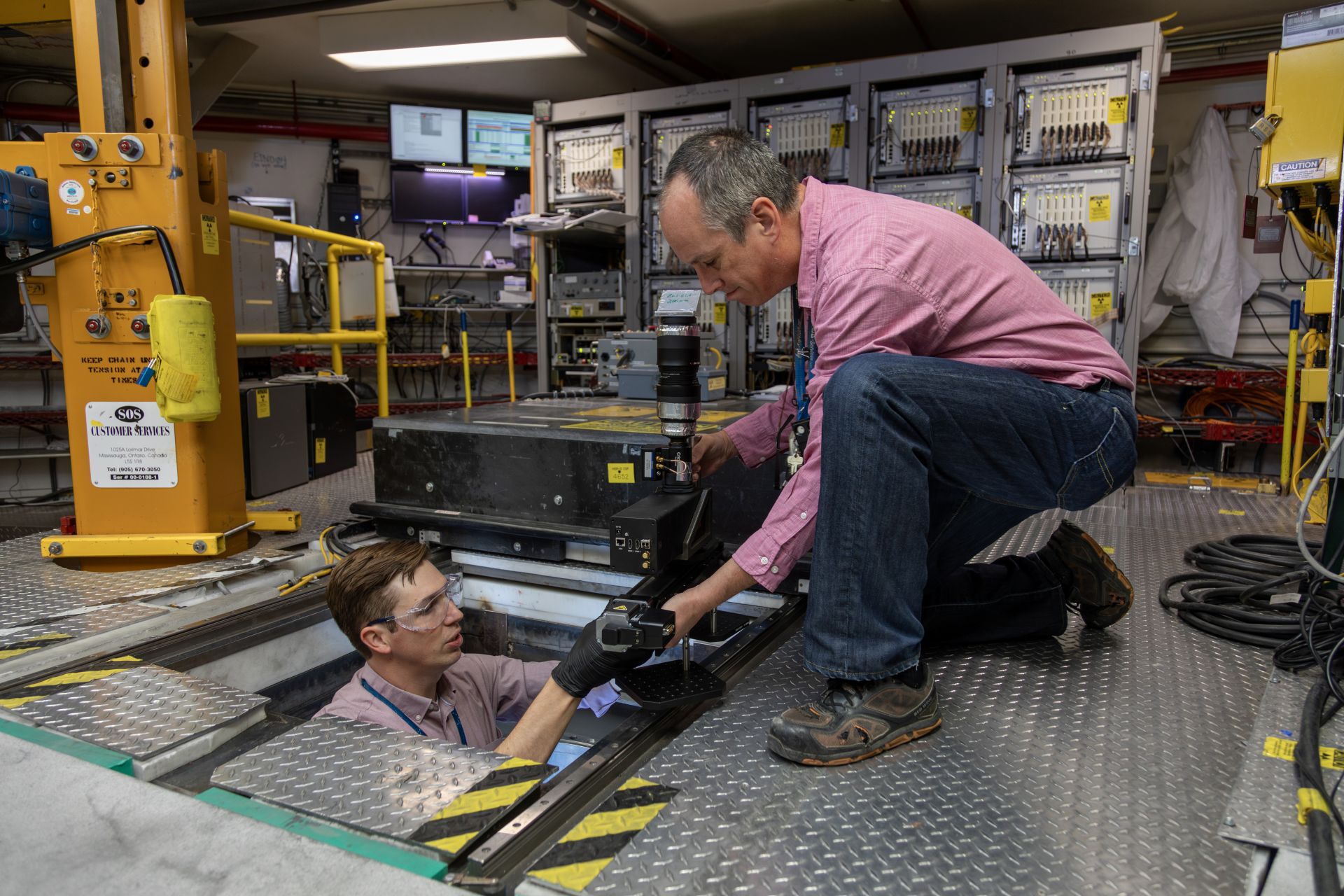
Beamline scientists installing a compact version of the LumaCam on Flight Path 4 (HIPPO). Funding to establish this imaging capability on Flight Path 4 was provided by the Nuclear Science User Facility (NSUF) program. The first simultaneous diffraction and imaging measurements of highly irradiated nuclear fuels were successfully conducted in February 2024. (Photo: LANL)
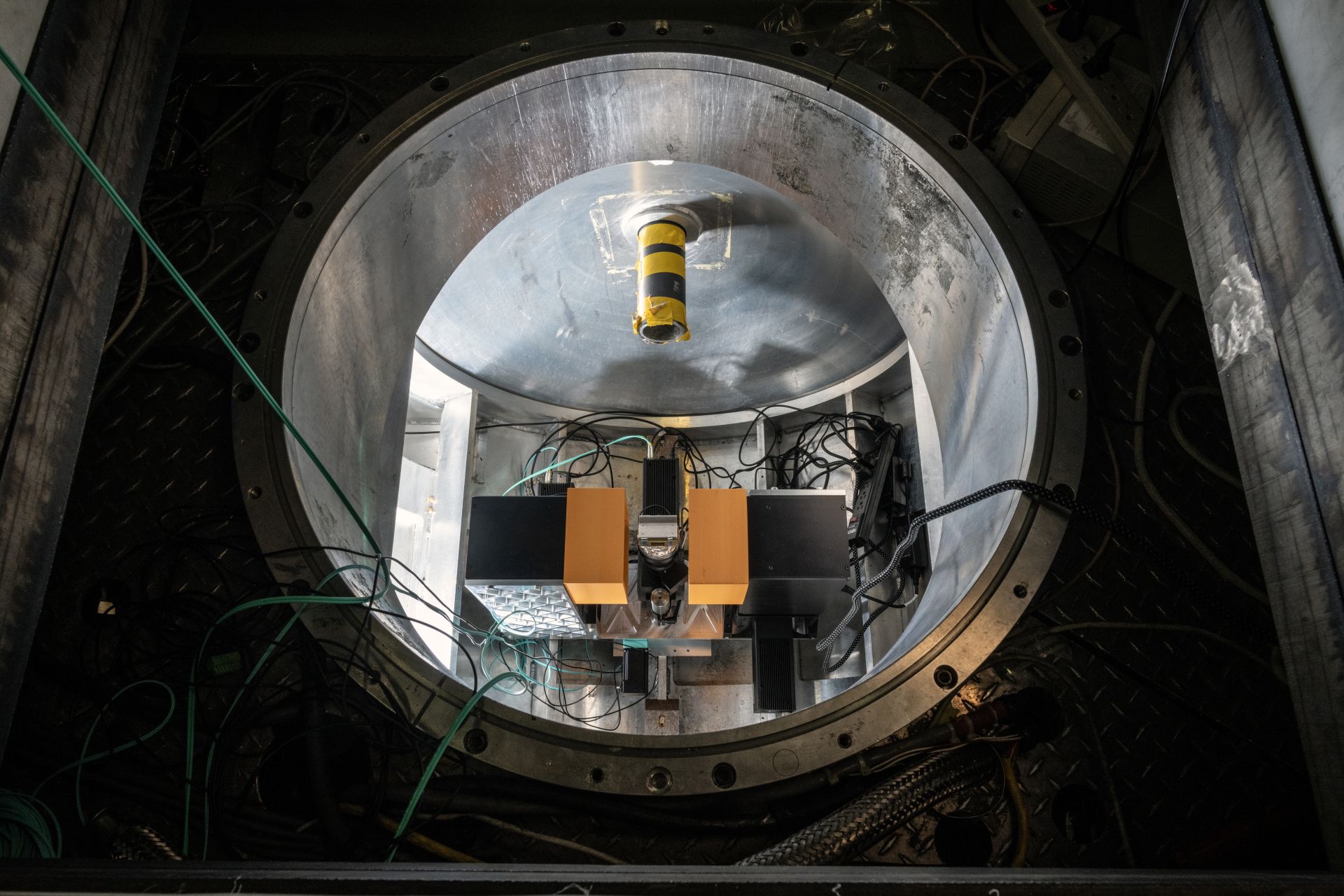
A top-down view of Flight Path 4 (HIPPO) at the Lujan Center, revealing the detector chamber housing over 1,200 helium-3 neutron detectors (not visible here). Recently, researchers installed an advanced neutron imaging system, known as a LumaCam, on this flight path to enable simultaneous time-of-flight neutron diffraction and energy-resolved neutron imaging. Alongside a LumaCam operating in transmission mode for imaging, researchers are also exploring the use of these systems in diffraction mode as a potential replacement for helium-3 detectors, paving the way for innovative advancements in neutron scattering flight path capabilities and enhancing the versatility of HIPPO for cutting-edge materials research. (Photo: LANL)
While NRI is a primary focus of ERNI, BEI is a standout capability of HIPPO. Recognized as a well-established time-of-flight neutron diffractometer, HIPPO has been upgraded with neutron imaging capabilities, enabling simultaneous imaging and diffraction measurements. This integration is a game changer, as it allows researchers to combine the spatial resolution of imaging with the sensitivity of bulk diffraction measurements. Here, neutrons diffracting off crystalline lattice planes create complementary signals in imaging and diffraction: While diffraction detects an increase in signal from neutrons scattered out of the beam, imaging records a corresponding decrease in beam intensity. Using bulk diffraction measurements like phase fractions and texture, researchers can better constrain and interpret the transmission spectra recorded in each pixel during BEI. This synergy directly improves the accuracy and quality of spatially resolved microstructure, making HIPPO a powerful tool for delivering high-quality and comprehensive insights into material behavior under diverse conditions.
In addition to the flight paths at the Lujan Center, the WNR Facility operates a highly pulsed, unmoderated neutron imaging flight path, known as Flight Path 60-R. This flight path is optimized for imaging with MeV neutrons, which offer unique advantages for penetrating dense materials such as centimeters of steel or lead while enabling the identification of lighter elements like hydrogen, carbon, oxygen, and silicon. These capabilities make Flight Path 60-R particularly well-suited for technique development of nuclear waste examination, where internal contents can be characterized nondestructively even in the presence of heavy shielding. By utilizing time-of-flight techniques to determine neutron energy, researchers can leverage the unique isotope-specific structure within the cross sections of lighter elements to map the spatial distribution of elemental compositions within nuclear waste packages. This pulsed MeV neutron imaging modality, known as fast neutron resonance radiography, has been recently demonstrated to effectively identify and map residual water, potential toxic substances, and other light-element compounds within mock-up waste containers and other samples.
While it is not economically viable to transport spent fuel to LANSCE for characterization, the neutron imaging methodologies refined on Flight Path 60-R are laying the groundwork for potential on-site analysis using compact short-pulsed neutron sources in the future. This cutting-edge nondestructive imaging technique would enable safer and more efficient localized waste evaluations at reactor sites by providing detailed insights into the internal structure and composition of waste assemblies, significantly reducing the potential need to open containers for further examination—a process that is both costly and logistically challenging.
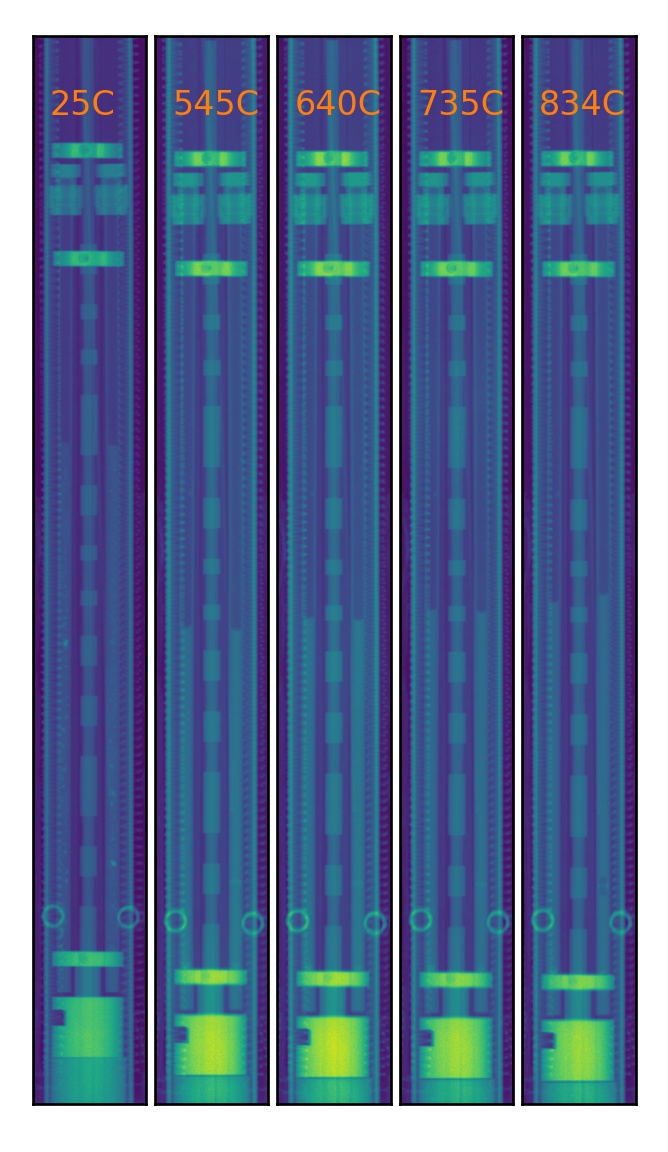
Five neutron attenuation images of NaCl+PuClx salt mixtures loaded into the furnace, taken at various temperatures ranging from ambient to approximately 850°C. These images capture the volumetric expansion of the samples as the temperature increases, highlighting the ability of neutron imaging to provide high-quality density measurements, as researchers can monitor the sample material in real time throughout the experiment. (Image: LANL)
While advanced neutron imaging is a focus, LANSCE continues to leverage traditional radiographic methods to address the needs of the nuclear energy sector. A prime example is the application of neutron imaging to study the thermophysical properties of molten salts, which are critical to advanced nuclear and energy systems. Achieving the high temperatures required for such studies necessitates the use of bulky furnaces while the aggressive chemistry requires substantial containment, making neutron imaging particularly advantageous. Neutrons not only offer high penetrability through dense containment materials but also provide sensitive contrast that highlights fluoride- or chloride-based salts with exceptional clarity. Using specialized setups, researchers at LANSCE have developed neutron imaging systems to remotely and nondestructively measure key thermophysical properties such as density and viscosity as a function of temperature in actinide-bearing chloride-based molten salts.
One major advantage of using imaging-based techniques is the ability to visually monitor the samples throughout the measurement process. This constant “eye” on the sample allows researchers to assess the status of the molten salt samples in real time, ensuring the integrity of the experiment. For instance, researchers can detect and address issues, such as bubbles forming within the melt, that could otherwise compromise the quality of the measurements and compromise the resulting data. Additionally, neutron imaging techniques often require lesser amounts of material compared to traditional thermophysical measurements. For example, density measurements of molten salt capillaries using neutron imaging often require less than 10 grams of sample material. This minimal material requirement and the immediate feedback on sample integrity make neutron imaging a highly valuable tool in advancing the understanding of complex molten salt systems, especially in the context of actinide-bearing materials in extreme environments.
To date, the molten salt research team at LANSCE has successfully performed and published several density measurements on uranium-based chloride systems, demonstrating the effectiveness of neutron imaging for these challenging materials. Additionally, the team has worked with industry partners through GAIN (Gateway for Accelerated Innovation in Nuclear) vouchers to measure the densities of several plutonium-based chloride systems. This collaboration underscores the growing role of neutron imaging in thermophysical property measurements within the molten salt reactor community.
Building a community around neutron imaging and materials for nuclear energy
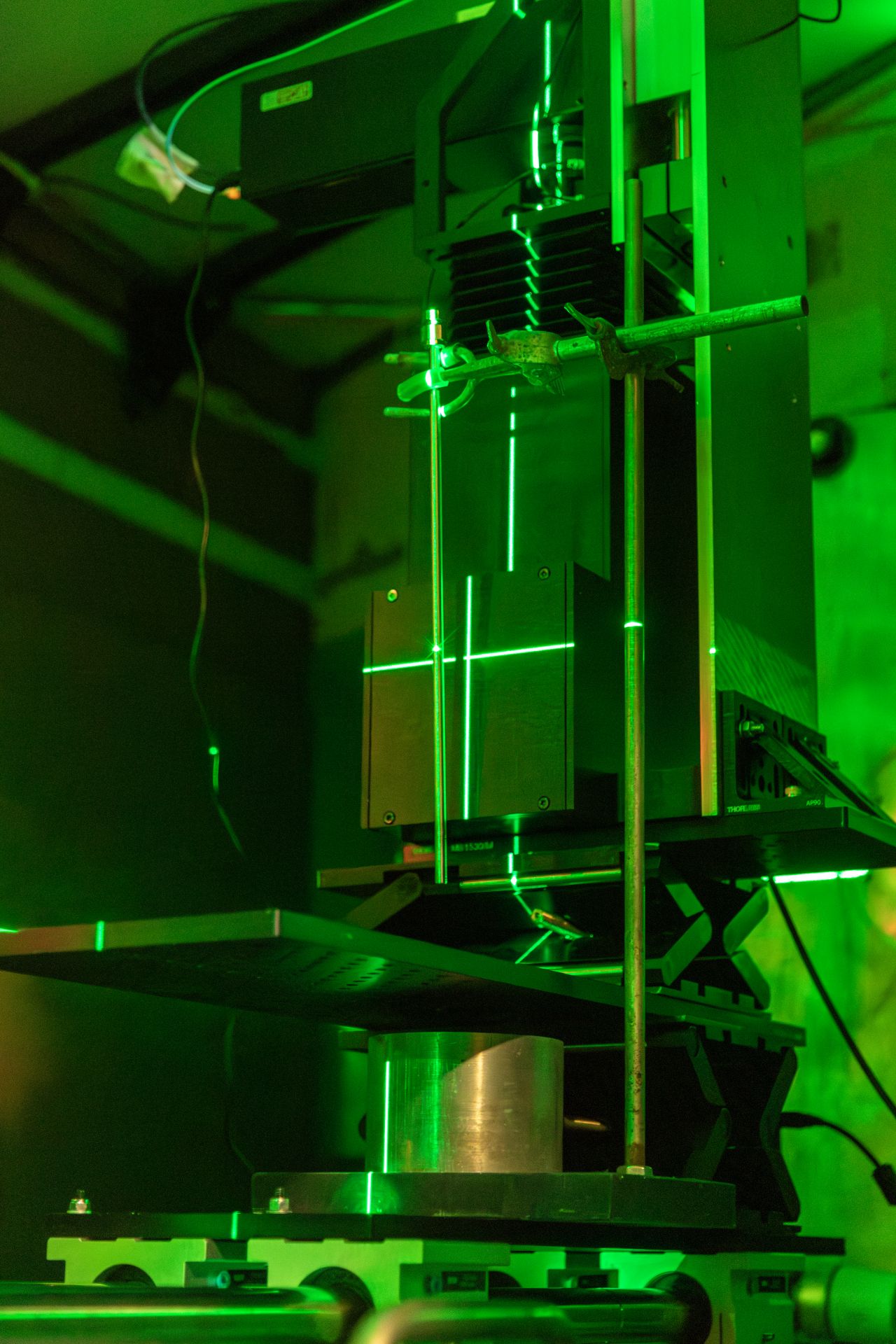
Advances in energy-resolved neutron imaging have been enabled by state-of-the-art camera technologies, such as those employing event-mode imaging sensors like Timepix. Commercial systems developed by companies like Amsterdam Scientific and LoskoVision provide highly configurable and user-friendly solutions for neutron imaging. Shown here is one such camera system installed on Flight Path 5 (ERNI) at the Lujan Center, capable of supporting advanced techniques such as Bragg-edge imaging and neutron resonance imaging. These systems, known as LumaCams, are now deployed across all neutron imaging flight paths at LANSCE, offering unparalleled capabilities for high-resolution, energy-resolved imaging for material science. (Photo: LANL)
The application of advanced neutron imaging techniques for studying material properties, isotopic compositions, and thermophysical properties in nuclear materials is expanding. With many of these techniques still under development, this interdisciplinary community—at the intersection of neutron imaging, materials science, and nuclear energy—is at a pivotal juncture. Continued progress in neutron imaging for nuclear material science will require collaborative efforts to refine imaging methodologies, improve instrumentation, and engage the community to drive innovative applications tailored to the unique material challenges of nuclear energy systems. By fostering a robust community centered around neutron imaging, we can accelerate material development to meet the needs of next-generation reactors.
To those interested in joining this burgeoning community or exploring the potential of neutron imaging for their own research, there are several opportunities to collaborate and propose new ideas at LANSCE. Each year, the material science program at LANSCE issues a proposal call in early spring, inviting researchers from around the world to submit ideas for measurements on the beamlines at the Lujan Center or the WNR Facility. Proposals are reviewed and awarded based on merit and available funding, offering a unique opportunity to access world-class neutron facilities. Additionally, LANSCE is a Nuclear Science User Facility (NSUF) partner, providing researchers with access to funding specifically for neutron scattering experiments on post-irradiated materials. This opportunity leverages LANSCE’s unique position as a facility that combines world-class neutron scattering capabilities with the authorization and infrastructure to handle hazardous and highly irradiated materials. Researchers can perform state-of-the-art post-irradiation examination measurements on highly irradiated materials.
For further information or to discuss potential ideas, researchers are encouraged to reach out directly to the flight path scientists listed on the LANSCE website, lansce.lanl.gov/. Together, we can continue to push the boundaries of neutron imaging and its applications in nuclear energy.
Alexander Long is a staff scientist at Los Alamos National Laboratory specializing in neutron imaging applied to material characterization. He serves as the instrument scientist for multiple neutron imaging beamlines at LANSCE, and his research focuses on developing advanced neutron imaging techniques to study the properties of materials for next-generation nuclear reactors.
Sven Vogel is a staff scientist at Los Alamos National Laboratory with expertise in neutron diffraction and imaging techniques. He serves as the beamline scientist for Flight Path 4 (HIPPO) at LANSCE, and his research spans topics including deformation of metals and alloys, diffusion pathways in battery materials, and the crystal- and microstructure structure evolution of nuclear fuels at temperature.